Predictive factors of activity of anti-programmed death-1/programmed death ligand-1 drugs: immunohistochemistry analysis
Introduction
Lung cancer currently remains the leading cause to cancer-related deaths globally; each year 1.8 million people are diagnosed with lung cancer (1). Lung cancer has traditionally been classified into small cell lung cancer (SCLC) and non-small cell lung cancer (NSCLC), with latter accounting for about 85% of all cases. Despite development of clinical diagnostic techniques, approximately 75% of all the NSCLC cases are diagnosed at an advanced or metastatic stage, thus leading to poor prognosis. With limited successful treatment options, surgery remains the standard treatment in the early stages of the NSCLC; for aggressive NSCLC, first-line of treatment is chemotherapy.
In the past decade, there have been several studies highlighting the role of EGFR and its mutations in lung tumorigenesis and response to EGFR small-molecule inhibitors (2). Even though identification of treatable mutations and development of new molecular targeted agents, the number of patients with these aberrations with access to these medications remain less than 20% (3). Thus, the prognosis of NSCLC remains grim, with a 5-year overall survival of high-stage patients approximately of 1% (4). Obviously, there is great need for a novel therapeutic approach that could improve the outcome for these NSCLC patients.
During the past few decades, there have been great advances in the understanding of the interaction between immune system and tumor progression. As such, lack of immunological control has been recognized as one of the tenets of cancer. Currently, there is increasing evidence pointing towards the formation of a tumor immune microenvironment that fosters tumorigenesis. Tumor cells disrupt host immune checkpoints thus escaping immune surveillance and avoiding their elimination from the host immune system. Transformation of normal to malignant cell involves several genetic and epigenetic changes, which can produce neoantigens that could be recognized by host immune system, thus triggering the body’s immune response. Under normal physiological conditions, T-cells would recognize cancer cells as abnormal and populate cytotoxic T-lymphocytes (CTLs) at the site thus infiltrating and killing cancer cells in the process. However, cancer cells utilize several pathways to up-regulate negative signals blocking T-cell activation and induction of cell death, thus promoting tumor growth and eventual metastases. Increasing number of studies and clinical trials show that an immunotherapeutic approach to utilizing antibodies against immune checkpoint modulators can release inhibitory signals and facilitate antitumor activity of the immune system.
In the current review, we would include the PD-1/programmed death ligand-1 (PD-L1) signaling in lung cancer and current efforts to employ immunohistochemical analysis to predict clinical response to anti-programmed death-1 (anti-PD1) and anti-PD-L1 medications.
Tumor immunogenicity
Early trials with various vaccines and immunostimulatory agents such as interleukin-2 that induces CTL proliferation failed to achieve satisfactory therapeutic outcome (5). Only a limited subset of NSCLC patients benefited with moderate survival benefits. However, there were immune-related side effects (6) leading to the belief that lung cancer is insensitive to immunotherapy.
In contrast, recent clinical data indicate that lung cancer is a suitable candidate for immunotherapy; patients with increased tumor-infiltrating lymphocytes (TILs), CTLs, T-helper cells, dendritic cells (DCs) and natural killer (NK) cells, have been shown to have a better prognosis (7-9). Lung tumors have also been shown to have high population of immunosuppressive cells like T-regulatory cells (T-regs) and myeloid-derived suppressor cells (MDSCs) thus disrupting killer cell activity (10). In addition, lung cancer cells secrete cytokines, interleukins, prostaglandins and growth factors attenuating T-cell response (11-14). There is also decrease in the levels of MHC class I expression in lung tumors facilitating the escape from routine antigen processing and evading immune surveillance (15). There are new and exciting data showing an improved rate of clinical response to antibodies targeting immune checkpoints (16). One of the goals of such immune checkpoint therapy is to remove the inhibitory pathways that block antitumor T cell response. Thus, the mechanism differs from traditional therapies targeting tumor or tumor vasculature, and earlier immunostimulatory.
Currently, research work in the field of immune checkpoint therapy is mainly focused on PD-1 and its ligand PD-L1 (B7H1). PD-1/PD-L1 axis regulates T cell activation in the later stages of tumor development. Antibodies targeting the PD-1/PD-L1 pathway have shown clinical responses in various cancers including NSCLC (17,18).
PD-1 and PD-L1
PD-1 was initially discovered while characterizing the role of de novo RNA synthesis in the apoptotic cell death of murine thymocytes (19), and earlier studies showed that it is a type 1 trans-membrane protein belonging to extended CD28/CTLA-4 immunoglobulin family and encoded by the PDCD1 gene (20). PD-1 is one the most important inhibitory co-receptors expressed on activated T cells. The PD-1 molecule comprises of an extracellular IgV domain, a hydrophobic transmembrane region, and an intracellular domain containing potential phosphorylation sites that are located in the immune tyrosine-based inhibitory motif (ITIM) and immune receptor inhibitory tyrosine-based switch motif (ITSM). Earlier mutagenic studies have shown that activated switch motif (ITSM) is required for the inhibitory effect of PD-1 on active T cells (21). Like other inhibitory co-receptors PD-1 is expressed by activated T cells, along with B cells, monocytes, NK cells, DCs, TILs, and activated T-regs, facilitating the proliferation of T-reg and thus, impeding immune response (22,23).
The PD-1 receptor has two ligands, PD-L1 (B7-H1 or CD274) and PD-L2 (B7-DC or CD273), which are shared by a co-inhibitory receptor CD80 (B7-1) (24,25). PD-L1 is expressed upon resting T cells, B cells, macrophages, DCs, pancreatic islet cells and endothelial cells. On the other hand, PD-L2 has restricted tissue distribution and is expressed only on antigen-presenting cells (APC). These differences in tissue distribution pattern suggest that these two molecules have separate function in immune modulation. This restricted expression of PD-L2 to macrophages and DC is in line with its role in regulating T-cell priming; in contrast, broadly expressed PD-L1 is involved in protecting peripheral tissues from excess of inflammation and autoimmune pathologies. PD-L1 has been found to be overexpressed in a wide variety of cancers viz. melanoma, glioblastoma, multiple myeloma, leukemias, and gastric, renal cell, bladder, hepatocellular, cutaneous, and NSCLC (26-32). PD-L1 is heterogeneously expressed in cancer and is found to be located in the cytoplasm and plasma membrane of cancer cells (33). PD-L2 is also expressed by various tumor cells, including lung adenocarcinomas (34).
PD-L1 expression is induced by various proinflammatory molecules such as interferon-γ (IFN-γ), tumor necrosis factor-α (TNF-α), vascular endothelial growth factor (VEGF), granulocyte-macrophage colony-stimulating factor (GM-CSF), and cytokines like IL-10. The tumor microenvironment has activated T helper cells, which produce IFN-γ and TNF-α, and tumor stromal cells secreting VEGF and GM-CSF, thus upregulating PD-L1 expression in tumor cells and facilitating immune suppression. This phenomenon has been named “adaptive immune resistance” (33). IFN-γ-induced PD-L1 expression in human cholangiocytes is shown to be regulated microRNA-513 (35). mir-531 is complementary to the 3’-UTR of PD-L1 mRNA, thus it prevents its translation. In addition, expression of PD-L1 is also regulated by phosphatase and tension homolog deleted on chromosome ten (PTEN), a tumor suppressive gene. PTEN is often mutated in tumor cells, resulting in the activation PI3K/AKT pathway and downstream effector mTOR-S6K1 signaling cascade, which causes increased translation of PD-L1 mRNA and high expression of PD-L1 protein in the cancer cells (36,37). In melanoma, the higher levels of PD-L1 protein in tumor cells were found to be correlated to the CD8+ T-cells (38).
In tumor cells as well as in T cells, PD-L1 can act both as a ligand and receptor. PD-L1, in absence or unavailability of its receptor PD-1, is capable of stimulating T cells (39,40). Stimulatory signal for activating T cell is received through binding of CD28 surface molecule to CD80 and CD86 molecules expressed on the APCs, on the other hand, interaction of CTLA-4 with these molecules down regulates T cell activity (41). However, T cells can also express CD80 and its docking with PD-L1 could induce T cell tolerance, and at this point CD80 acts an additional T cell counter receptor for PD-L1 with inhibitory function (24).
PD-1/PD-L1 signaling pathway
Under normal physiological conditions, PD-1 regulates the activity of effector T-cells in peripheral tissues in response to infection. This is a critical step in protecting human body against tissue damage upon the activation of immune system. PD-L1 and PD-L2 expressed on the APC surface exert suppressive action via T-cell receptors. The binding of PD-L1 or PD-L2 leads to recruitment of tyrosine phosphatases generating an inhibitory signal that blocks downstream effects of PI3K/Akt signaling resulting in cell cycle arrest and suppressed T-cell activation (21,42,43). However, when cancer cells are attacked by immune system they start overexpressing PD-L1 and PD-L2, which bind to PD-1 receptors on T-cells suppressing them and in the process resulting in tumor immune escape (44).
In dynamic tumor microenvironment, PD-1 and its ligand PD-L1 down regulate T-cell response by various mechanisms. Recently, Duraiswamy & coworkers showed that overexpression of PD-L1 on tumor cells, tumor-associated macrophages, DCs, and MDSCs positively correlated with the exhaustion of TILs in tumor (45). PD-L1 down regulates phospho-Erk and increases PTEN causing inhibition of Akt/mTOR/S6 signaling pathway, hence, promoting generation of induced T-regs, which restrain the activity of effector CD8 T cell (23). Also, earlier reports show that PD-1 induces cell death in activated T cells via inhibiting PI3K activation leading to down regulation of anti-apoptotic protein Bcl-xL (46). Anti-PD-L1 antibody has been shown to decrease the apoptosis of T cells, and could help in achieving tumor immunity. In addition, PD-1 could also inhibit phosphorylation of PKC-θ, which is required for production growth stimulatory IL-2, thus resulting in cell cycle arrest and blocking T cell proliferation (47).
PD-L1 is overexpressed in various malignancies viz. melanoma, glioblastoma, and renal, gastric, head and neck squamous cell, colon, pancreas, breast, cervical, ovarian, and lung carcinoma (31,32,48-57). PD-L1 protein expression is a poor prognostic biomarker in NSCLC and found to be associated with tumor differentiation (58). Studies have shown that lung cancer patients with EGFR mutations have higher levels of PD-L1 expression than the wild-type samples and high PD-L1 expression is associated with the presence of EGFR mutation (59). In lung cancers not harboring EGFR mutation, the PD-L1 over expression conveys poor prognosis (60). Also, a recent study revealed a direct link between tumor-related genes and imbalance of immune regulation. The authors showed that either EML4-ALK fusion gene or activating mutations of the EGFR upregulated PD-L1 expression in NSCLC cell lines by activating PI3K-AKT and MEK-ERK signaling pathways (61). There was also a direct correlation between the levels of EML4-ALK and PD-L1 expression in NSCLC tissue specimens.
Agents targeting PD-1/PD-L1
Currently, several immune-oncology agents targeting PD-1/PD-L1 are being developed. These novel promising immune checkpoint blockers have shown benefits in recent clinical trials, including the NSCLC patients.
As described above, PD-1 is an immunoregulatory receptor that is expressed by activated T cells (62). Although not all the cells expressing PD-1 are exhausted, postulating a theory that blocking PD-1 can restore the function of T cells (63). Nivolumab (BMS-936558 or MDX1106b) is a human IgG4 antibody against PD-1, and lacks detectable antibody-dependent cellular toxicity (ADCC). In phase I clinical trials Nivolumab showed remarkable regression in various tumors, including NSCLC (64), and in a recent study, previously treated metastatic squamous-cell NSCLC patients had a significantly better overall survival, response rate, and progression-free survival with Nivolumab than with Docetaxel (65). In March 2015, by the United States Food and Drug Administration (FDA) approved nivolumab to be used in treating patients with metastatic squamous NSCLC that progressed on or after platinum-based chemotherapy.
Pembrolizumab (MK-3475) is another highly selective anti-PD-1 humanized monoclonal IgG4 kappa isotype antibody that contains mutation at C228P designed to prevent Fc-mediated cytotoxicity. It can disrupt the engagement of PD-1 and PD-L1, resulting tumor recognition by cytotoxic T cells. In a recent phase-I trial, Pembrolizumab showed antitumor activity and had an acceptable toxicity profile in patients with advanced NSCLC (66).
Another strategy of attenuating PD-1 and PD-L1 signaling cascade is by anti-PD-L1 antibody binding with PD-L1 molecules. Targeting PD-L1 might also result in less treatment-related toxicity partly by instigating selective immune response in the tumor micro milieu. BMS-936559/MDX1105 and MPDL3280A are anti-PD-L1 monoclonal antibodies reacting specifically with PD-L1, and preventing its docking with PD-1 and CD80. BMS-936559/MDX1105 is a high affinity, fully humanized IgG4 antibody, whereas MPDL3280A is an engineered, human monoclonal IgG1 antibody with modified Fc component so as not to activate ADCC. In a multicentric, dose-escalation phase I trial in advanced solid tumors that included 75 NSCLC patients, 6–17% of objective response rate (ORR) and prolonged stabilization of disease (12–41% at 24 weeks) were observed (67). In a phase I trial of MPDL3280A, 175 patients (with 53 NSCLC cases) were enrolled and had a 21% ORR with 39% having stable disease (68).
Predictive biomarkers for immune-modulatory agents
With the development of targeted and more personalized therapies, there is a need to identify predictive biomarkers with which to select patients for treatment with a particular agent. In recent years, there have been various reports exploring PD-L1 expression as a criterion for selecting anti-PD-1/PD-L1 therapies (69).
The initial phase I Nivolumab trial in 296 patients reported 18% ORR in NSCLC patients reported (17 of 76 patients), 28% among patients with melanoma (26 of 94 patients), and 27% among patients with renal-cell carcinoma (9 of 33 patients). Immunohistochemical analysis on pretreatment tumor samples revealed that patients with PD-L1 positive (≥5% tumor cells with PD-L1 expression) tumor specimens had an excellent 36% ORR. On the other hand, patients with PD-L1 negative tumor specimens did not have any objective response (70). Similarly, in a phase I study with anti-PD-L1 antibody (MPDL3280A) across various solid tumors, responses (as evaluated by RECIST, version 1.1) were observed in patients with tumors expressing high levels of PD-L1, especially when PD-L1 was expressed by tumor-infiltrating immune cells (68). However, in a randomized, multicentric, phase-3 study that compared Nivolumab monotherapy with Docetaxel monotherapy in patients with advanced squamous-cell NSCLC in whom the disease progressed during or after one prior platinum-containing chemotherapy regimen authors found that the PD-L1 expression was neither prognostic nor predictive of therapeutic benefit (65). These conflicting reports call into question the potential role of PD-L1 as a predictive biomarker to select a patient subgroup for anti-PD-1/PD-L1 therapy.
These contradicting observations of PD-L1 immunoexpression and possible correlation with tumor response may be attributed to the use of various antibodies for detecting PD-L1 by immunohistochemistry (IHC) in clinical trials. Initial report from Topalian used a murine antihuman PD-L1 monoclonal antibody 5H1 (70); Herbst et al., used a an anti-human PD-L1 rabbit monoclonal antibody clone SP142 from Ventana, Tucson (68), whereas, Brahmer and coworkers used a rabbit monoclonal, antihuman PD-L1 antibody (clone 28–8, Abcam) (65). In an in-depth review by Teixidó et al. they consolidated an extensive list of PD-L1 antibodies used to-date for immunohistochemically detecting PD-L1 expression in various studies (69). All three studies examined PD-L1 expression in formalin-fixed paraffin-embedded (FFPE) tissue sections, however tissue fixation, epitope stability and processing could impact the outcome of immunohistochemical reactions (71).
Whereas frozen specimens can be stained very specifically, FFPE material could result in weaker staining and high background. Such observations have been confirmed by others using PD-L1 monoclonal antibody 5H1 (33,72). Unmasking of the epitopes that are masked by protein linkage during formalin fixation is performed via heat-induced epitope retrieval (HIER) using buffer solutions [citrate buffer (pH 6), ethylenediaminetetraacetic acid (EDTA) buffer (pH 9), and Tris buffer (pH 10)], which includes high-temperature heat-induced cleavage of the protein—protein cross-links produced during fixation of tissues in neutral-buffered formalin (73). In various studies published so far there is limited information on methodology for PD-L1 staining; specifically whether a low- or high-pH antigen retrieval buffer was used is unclear. However, it has been documented that HIER with a citrate buffer (pH 6) can produce results superior to those obtained with buffers with a higher pH (74).
In our laboratory, we have performed immunohistochemical analysis of PD-L1 protein expression in FFPE sections of melanoma lesions using a rabbit monoclonal antibody against PD-L1 {clone EPR1161 [2] Abcam, Cambridge, MA, USA}at dilution 1:100, after HIER with citrate buffer (pH 6). We have observed a predominantly membranous pattern along with occasional cytoplasmic staining (Figure 1A). For PD-1 immunostaining, after HIER using citrate buffer (pH 6), we have used mouse monoclonal antibody against PD-1 of human origin [clone (NAT105); Abcam, Cambridge, MA, USA] at dilution 1:250, and have observed only membranous staining in the lymphocytes (Figure 1B). For both these antibodies we have used labeled streptavidin biotin (LSAB) method (Dako, Carpinteria, CA, USA), which compared to ABC method results in less background staining.
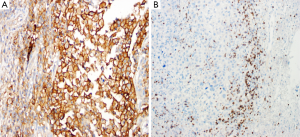
Furthermore, even if the immunohistochemical study is correctly performed, it may be difficult to determine a cutoff that defines a clinically significant positive and predictive value. Furthermore, the goal is to determine which patients will likely benefit of the treatment. Although most studies have regarded PD-L1 membranous labeling of the tumor cells as the most significant one, PD-L1 expression can be detected by IHC in both tumor cells and in immune effector cells (tumor infiltrating immune cells) (17,70,75).
Regarding evaluation of the immunohistochemical slides, there may be different cut-offs, such as ≥1%, ≥5%, or ≥10% of cells (68); ≥5% cells (70); or 1%, 5% or 10% cells in at least 100 evaluable tumor cells (65). A possible pitfall in some of these studies is the fact that PD-L1 is heterogeneously expressed in tumor cells and thus examination of tissue microarrays or even just a small biopsy of a large tumor may not project an accurate picture of PD-L1 pattern of expression.
In addition to evaluating PD-L1 expression, other biomarkers that play a role in antitumor response educed by immunotherapy may also be identified through genetic analysis of tumor cells. In patients treated with MPDL3280A, high expression of CTLA4 (an important regulator during T-cell expansion) in pre-treatment specimens was observed to correlate with response to therapy (68).
Conclusions
Based upon the studies reported so far, use of PD-L1 expression as a predictive biomarker for anti-PD-1/PD-L1 immunotherapy remains a possible method for selection or exclusion of patients for treatment (28). However, due to the existence of various available antibodies for detecting PD-L1 expression with different scoring systems, epitope stability, usage of varying sample types viz. small biopsies, cytology specimens and large tumor samples, and intra- and inter-laboratory reproducibility, it remains to be determined the optimal, standardized method for clinical trials.
Acknowledgements
Funding: This work was supported in part by the UT MD Anderson Cancer Center SPORE in Melanoma (P50 CA093459) funded from the NCI, Aim at Melanoma Foundation and the Miriam & Jim Mulva Research Funds, and the MD Anderson CCSG grant (P30 CA016672) funded from the NIH National Cancer Institute.
Footnote
Conflicts of Interest: The authors have no conflicts of interest to declare.
References
- Torre LA, Bray F, Siegel RL, et al. Global cancer statistics, 2012. CA Cancer J Clin 2015;65:87-108. [PubMed]
- Sharma SV, Bell DW, Settleman J, et al. Epidermal growth factor receptor mutations in lung cancer. Nat Rev Cancer 2007;7:169-81. [PubMed]
- Cardarella S, Ortiz TM, Joshi VA, et al. The introduction of systematic genomic testing for patients with non-small-cell lung cancer. J Thorac Oncol 2012;7:1767-74. [PubMed]
- Brundage MD, Davies D, Mackillop WJ. Prognostic factors in non-small cell lung cancer: a decade of progress. Chest 2002;122:1037-57. [PubMed]
- Jansen RL, Slingerland R, Goey SH, et al. Interleukin-2 and interferon-alpha in the treatment of patients with advanced non-small-cell lung cancer. J Immunother (1991) 1992;12:70-3. [PubMed]
- Murala S, Alli V, Kreisel D, et al. Current status of immunotherapy for the treatment of lung cancer. J Thorac Dis 2010;2:237-44. [PubMed]
- Al-Shibli KI, Donnem T, Al-Saad S, et al. Prognostic effect of epithelial and stromal lymphocyte infiltration in non-small cell lung cancer. Clin Cancer Res 2008;14:5220-7. [PubMed]
- Kawai O, Ishii G, Kubota K, et al. Predominant infiltration of macrophages and CD8(+) T Cells in cancer nests is a significant predictor of survival in stage IV nonsmall cell lung cancer. Cancer 2008;113:1387-95. [PubMed]
- Miotto D, Lo Cascio N, Stendardo M, et al. CD8+ T cells expressing IL-10 are associated with a favourable prognosis in lung cancer. Lung Cancer 2010;69:355-60. [PubMed]
- Nagaraj S, Youn JI, Gabrilovich DI. Reciprocal relationship between myeloid-derived suppressor cells and T cells. J Immunol 2013;191:17-23. [PubMed]
- Shimizu K, Nakata M, Hirami Y, et al. Tumor-infiltrating Foxp3+ regulatory T cells are correlated with cyclooxygenase-2 expression and are associated with recurrence in resected non-small cell lung cancer. J Thorac Oncol 2010;5:585-90. [PubMed]
- Jadus MR, Natividad J, Mai A, et al. Lung cancer: a classic example of tumor escape and progression while providing opportunities for immunological intervention. Clin Dev Immunol 2012;2012:160724.
- Yao Z, Fenoglio S, Gao DC, et al. TGF-beta IL-6 axis mediates selective and adaptive mechanisms of resistance to molecular targeted therapy in lung cancer. Proc Natl Acad Sci U S A 2010;107:15535-40. [PubMed]
- Baratelli F, Lee JM, Hazra S, et al. PGE(2) contributes to TGF-beta induced T regulatory cell function in human non-small cell lung cancer. Am J Transl Res 2010;2:356-67. [PubMed]
- Ramnath N, Tan D, Li Q, et al. Is downregulation of MHC class I antigen expression in human non-small cell lung cancer associated with prolonged survival? Cancer Immunol Immunother 2006;55:891-9. [PubMed]
- Drake CG, Lipson EJ, Brahmer JR. Breathing new life into immunotherapy: review of melanoma, lung and kidney cancer. Nat Rev Clin Oncol 2014;11:24-37. [PubMed]
- Powles T, Eder JP, Fine GD, et al. MPDL3280A (anti-PD-L1) treatment leads to clinical activity in metastatic bladder cancer. Nature 2014;515:558-62. [PubMed]
- Topalian SL, Drake CG, Pardoll DM. Targeting the PD-1/B7-H1(PD-L1) pathway to activate anti-tumor immunity. Curr Opin Immunol 2012;24:207-12. [PubMed]
- Ishida Y, Agata Y, Shibahara K, et al. Induced expression of PD-1, a novel member of the immunoglobulin gene superfamily, upon programmed cell death. EMBO J 1992;11:3887-95. [PubMed]
- Ceeraz S, Nowak EC, Noelle RJ. B7 family checkpoint regulators in immune regulation and disease. Trends Immunol 2013;34:556-63. [PubMed]
- Riley JL. PD-1 signaling in primary T cells. Immunol Rev 2009;229:114-25. [PubMed]
- Keir ME, Butte MJ, Freeman GJ, et al. PD-1 and its ligands in tolerance and immunity. Annu Rev Immunol 2008;26:677-704. [PubMed]
- Francisco LM, Salinas VH, Brown KE, et al. PD-L1 regulates the development, maintenance, and function of induced regulatory T cells. J Exp Med 2009;206:3015-29. [PubMed]
- Butte MJ, Keir ME, Phamduy TB, et al. Programmed death-1 ligand 1 interacts specifically with the B7-1 costimulatory molecule to inhibit T cell responses. Immunity 2007;27:111-22. [PubMed]
- Butte MJ, Peña-Cruz V, Kim MJ, et al. Interaction of human PD-L1 and B7-1. Mol Immunol 2008;45:3567-72. [PubMed]
- Thompson RH, Gillett MD, Cheville JC, et al. Costimulatory B7-H1 in renal cell carcinoma patients: Indicator of tumor aggressiveness and potential therapeutic target. Proc Natl Acad Sci U S A 2004;101:17174-9. [PubMed]
- Boland JM, Kwon ED, Harrington SM, et al. Tumor B7-H1 and B7-H3 expression in squamous cell carcinoma of the lung. Clin Lung Cancer 2013;14:157-63. [PubMed]
- Velcheti V, Schalper KA, Carvajal DE, et al. Programmed death ligand-1 expression in non-small cell lung cancer. Lab Invest 2014;94:107-16. [PubMed]
- Cooper WA, Tran T, Vilain RE, et al. PD-L1 expression is a favorable prognostic factor in early stage non-small cell carcinoma. Lung Cancer 2015;89:181-8. [PubMed]
- Huang Y, Zhang SD, McCrudden C, et al. The prognostic significance of PD-L1 in bladder cancer. Oncol Rep 2015;33:3075-84. [PubMed]
- Kakavand H, Vilain RE, Wilmott JS, et al. Tumor PD-L1 expression, immune cell correlates and PD-1+ lymphocytes in sentinel lymph node melanoma metastases. Mod Pathol 2015;28:1535-44. [PubMed]
- Nduom EK, Wei J, Yaghi NK, et al. PD-L1 expression and prognostic impact in glioblastoma. Neuro Oncol 2015. [Epub ahead of print]. [PubMed]
- Taube JM, Anders RA, Young GD, et al. Colocalization of inflammatory response with B7-h1 expression in human melanocytic lesions supports an adaptive resistance mechanism of immune escape. Sci Transl Med 2012;4:127ra37.
- Zhang Y, Wang L, Li Y, et al. Protein expression of programmed death 1 ligand 1 and ligand 2 independently predict poor prognosis in surgically resected lung adenocarcinoma. Onco Targets Ther 2014;7:567-73. [PubMed]
- Gong AY, Zhou R, Hu G, et al. MicroRNA-513 regulates B7-H1 translation and is involved in IFN-gamma-induced B7-H1 expression in cholangiocytes. J Immunol 2009;182:1325-33. [PubMed]
- Parsa AT, Waldron JS, Panner A, et al. Loss of tumor suppressor PTEN function increases B7-H1 expression and immunoresistance in glioma. Nat Med 2007;13:84-8. [PubMed]
- Crane CA, Panner A, Murray JC, et al. PI(3) kinase is associated with a mechanism of immunoresistance in breast and prostate cancer. Oncogene 2009;28:306-12. [PubMed]
- Spranger S, Spaapen RM, Zha Y, et al. Up-regulation of PD-L1, IDO, and T(regs) in the melanoma tumor microenvironment is driven by CD8(+) T cells. Sci Transl Med 2013;5:200ra116.
- Dong H, Zhu G, Tamada K, et al. B7-H1, a third member of the B7 family, co-stimulates T-cell proliferation and interleukin-10 secretion. Nat Med 1999;5:1365-9. [PubMed]
- Azuma T, Yao S, Zhu G, et al. B7-H1 is a ubiquitous antiapoptotic receptor on cancer cells. Blood 2008;111:3635-43. [PubMed]
- Chen L. Co-inhibitory molecules of the B7-CD28 family in the control of T-cell immunity. Nat Rev Immunol 2004;4:336-47. [PubMed]
- Okazaki T, Honjo T. PD-1 and PD-1 ligands: from discovery to clinical application. Int Immunol 2007;19:813-24. [PubMed]
- Sznol M, Chen L. Antagonist antibodies to PD-1 and B7-H1 (PD-L1) in the treatment of advanced human cancer--response. Clin Cancer Res 2013;19:5542. [PubMed]
- Zou W, Chen L. Inhibitory B7-family molecules in the tumour microenvironment. Nat Rev Immunol 2008;8:467-77. [PubMed]
- Duraiswamy J, Freeman GJ, Coukos G. Therapeutic PD-1 pathway blockade augments with other modalities of immunotherapy T-cell function to prevent immune decline in ovarian cancer. Cancer Res 2013;73:6900-12. [PubMed]
- Dong H, Strome SE, Salomao DR, et al. Tumor-associated B7-H1 promotes T-cell apoptosis: a potential mechanism of immune evasion. Nat Med 2002;8:793-800. [PubMed]
- Sheppard KA, Fitz LJ, Lee JM, et al. PD-1 inhibits T-cell receptor induced phosphorylation of the ZAP70/CD3zeta signalosome and downstream signaling to PKCtheta. FEBS Lett 2004;574:37-41. [PubMed]
- Nomi T, Sho M, Akahori T, et al. Clinical significance and therapeutic potential of the programmed death-1 ligand/programmed death-1 pathway in human pancreatic cancer. Clin Cancer Res 2007;13:2151-7. [PubMed]
- Thompson RH, Kuntz SM, Leibovich BC, et al. Tumor B7-H1 is associated with poor prognosis in renal cell carcinoma patients with long-term follow-up. Cancer Res 2006;66:3381-5. [PubMed]
- Thompson RH, Kwon ED. Significance of B7-H1 overexpression in kidney cancer. Clin Genitourin Cancer 2006;5:206-11. [PubMed]
- Wu C, Zhu Y, Jiang J, et al. Immunohistochemical localization of programmed death-1 ligand-1 (PD-L1) in gastric carcinoma and its clinical significance. Acta Histochem 2006;108:19-24. [PubMed]
- Vassilakopoulou M, Avgeris M, Velcheti V, et al. Evaluation of PD-L1 Expression and Associated Tumor-Infiltrating Lymphocytes in Laryngeal Squamous Cell Carcinoma. Clin Cancer Res 2015. [Epub ahead of print].
- Mezache L, Paniccia B, Nyinawabera A, et al. Enhanced expression of PD L1 in cervical intraepithelial neoplasia and cervical cancers. Mod Pathol 2015;28:1594-602. [PubMed]
- Sabbatino F, Villani V, Yearley JH, et al. PD-L1 and HLA Class I Antigen Expression and Clinical Course of the Disease in Intrahepatic Cholangiocarcinoma. Clin Cancer Res 2015. [Epub ahead of print]. [PubMed]
- Chen TC, Wu CT, Wang CP, et al. Associations among pretreatment tumor necrosis and the expression of HIF-1α and PD-L1 in advanced oral squamous cell carcinoma and the prognostic impact thereof. Oral Oncol 2015;51:1004-10. [PubMed]
- Thierauf J, Veit JA, Affolter A, et al. Identification and clinical relevance of PD-L1 expression in primary mucosal malignant melanoma of the head and neck. Melanoma Res 2015;25:503-9. [PubMed]
- Kim S, Kim MY, Koh J, et al. Programmed death-1 ligand 1 and 2 are highly expressed in pleomorphic carcinomas of the lung: Comparison of sarcomatous and carcinomatous areas. Eur J Cancer 2015;51:2698-707. [PubMed]
- Pan ZK, Ye F, Wu X, et al. Clinicopathological and prognostic significance of programmed cell death ligand1 (PD-L1) expression in patients with non-small cell lung cancer: a meta-analysis. J Thorac Dis 2015;7:462-70. [PubMed]
- Azuma K, Ota K, Kawahara A, et al. Association of PD-L1 overexpression with activating EGFR mutations in surgically resected nonsmall-cell lung cancer. Ann Oncol 2014;25:1935-40. [PubMed]
- Tang Y, Fang W, Zhang Y, et al. The association between PD-L1 and EGFR status and the prognostic value of PD-L1 in advanced non-small cell lung cancer patients treated with EGFR-TKIs. Oncotarget 2015;6:14209-19. [PubMed]
- Ota K, Azuma K, Kawahara A, et al. Induction of PD-L1 Expression by the EML4-ALK Oncoprotein and Downstream Signaling Pathways in Non-Small Cell Lung Cancer. Clin Cancer Res 2015;21:4014-21. [PubMed]
- Radziewicz H, Ibegbu CC, Fernandez ML, et al. Liver-infiltrating lymphocytes in chronic human hepatitis C virus infection display an exhausted phenotype with high levels of PD-1 and low levels of CD127 expression. J Virol 2007;81:2545-53. [PubMed]
- Barber DL, Wherry EJ, Masopust D, et al. Restoring function in exhausted CD8 T cells during chronic viral infection. Nature 2006;439:682-7. [PubMed]
- de Mello RA, Pousa I, Pereira D. Nivolumab for advanced squamous cell lung cancer: what are the next steps? Lancet Oncol 2015;16:234-5. [PubMed]
- Brahmer J, Reckamp KL, Baas P, et al. Nivolumab versus Docetaxel in Advanced Squamous-Cell Non-Small-Cell Lung Cancer. N Engl J Med 2015;373:123-35. [PubMed]
- Garon EB, Rizvi NA, Hui R, et al. Pembrolizumab for the treatment of non-small-cell lung cancer. N Engl J Med 2015;372:2018-28. [PubMed]
- Brahmer JR, Tykodi SS, Chow LQ, et al. Safety and activity of anti-PD-L1 antibody in patients with advanced cancer. N Engl J Med 2012;366:2455-65. [PubMed]
- Herbst RS, Soria JC, Kowanetz M, et al. Predictive correlates of response to the anti-PD-L1 antibody MPDL3280A in cancer patients. Nature 2014;515:563-7. [PubMed]
- Teixidó C, Karachaliou N, González-Cao M, et al. Assays for predicting and monitoring responses to lung cancer immunotherapy. Cancer Biol Med 2015;12:87-95. [PubMed]
- Topalian SL, Hodi FS, Brahmer JR, et al. Safety, activity, and immune correlates of anti-PD-1 antibody in cancer. N Engl J Med 2012;366:2443-54. [PubMed]
- Bussolati G, Leonardo E. Technical pitfalls potentially affecting diagnoses in immunohistochemistry. J Clin Pathol 2008;61:1184-92. [PubMed]
- Gadiot J, Hooijkaas AI, Kaiser AD, et al. Overall survival and PD-L1 expression in metastasized malignant melanoma. Cancer 2011;117:2192-201. [PubMed]
- Yamashita S. Heat-induced antigen retrieval: mechanisms and application to histochemistry. Prog Histochem Cytochem 2007;41:141-200. [PubMed]
- Ramos-Vara JA. Technical aspects of immunohistochemistry. Vet Pathol 2005;42:405-26. [PubMed]
- Tumeh PC, Harview CL, Yearley JH, et al. PD-1 blockade induces responses by inhibiting adaptive immune resistance. Nature 2014;515:568-71. [PubMed]